Published On September 21, 2018
AS AUGUST BEGINS ITS slow fade into September in Alaska’s tundra, Arctic ground squirrels (Spermophilus parryii) retreat into underground burrows to hibernate through the freezing winter. The squirrels’ metabolism slows to near-zero; their hearts beat and their lungs inflate only once per minute; and their body temperature drops as low as 27 degrees Fahrenheit. Their brains, normally greedy for oxygen and glucose, receive almost no blood. Yet Arctic ground squirrels will emerge in spring undamaged, after cooling and warming themselves numerous times throughout their frozen sojourn.
A human who underwent such changes would die within minutes. But some researchers believe that understanding how the squirrels survive these oscillations in their metabolism could lead to new strategies for treating people who are having a stroke, in which blood also stops circulating to the brain. “The blood flow is so low in these hibernating animals that you’d think they were having a stroke,” says Kelly Drew, a biochemist at the Institute of Arctic Biology at the University of Alaska Fairbanks, who has studied Arctic ground squirrel hibernation for more than 20 years. “But they have no signs of brain injury, which shows just how protective hibernation is.”
It protects them in another way, too, shielding ground squirrels and other hibernating animals—ranging in size from diminutive Syrian hamsters to cave bats to Madagascar’s fat-tailed dwarf lemur to massive grizzly bears—from harm when they warm up and blood flow resumes, which happens repeatedly during hibernation and again finally in the spring when outdoor conditions improve. That also seems relevant to what occurs during a human stroke. Only some of a stroke’s damage comes from the initial lack of blood, with a cascade of additional injuries occurring during “reperfusion,” when warm, oxygenated blood returns to the tissues and immune processes kick into high gear.
Millions of years of evolution have given hibernators this seemingly miraculous ability to survive the equivalent of a stroke and its aftermath more than 30 times each year, all without signs of injury or distress. Hannah Carey, a physiologist at the University of Wisconsin, Madison, is another scientist who believes that solving the mysteries of how that happens might lead to treatments that could help prevent or reduce the harm to people who have a stroke. That includes 800,000 Americans each year, of whom 130,000 die as a result. “If we can tease out what is so special about hibernators, we might be able to apply that to medical treatments in humans,” Carey says.
That quest to unravel what actually happens during hibernation isn’t an easy one. For starters, the most common animal models in human medical research—including rats, mice and zebrafish—don’t hibernate, so research on hibernators lacks the deep well of genetic and molecular data that has been built up from research on these model organisms. Moreover, some hibernators, such as the Arctic ground squirrel, don’t breed well in captivity, which means new research subjects must be captured from the wild.
Working within these constraints, scientists have discovered that hibernation is far more complex than a long winter’s nap. It affects every organ system in the body and doesn’t have a simple on/off switch that can be flipped at will. Still, comprehending even small aspects of hibernation could ultimately lead to much-needed new stroke therapies, according to Sandy Martin, a molecular biologist at the University of Colorado. “It’s not really that far-fetched to think that humans could make use of these mechanisms,” she says.
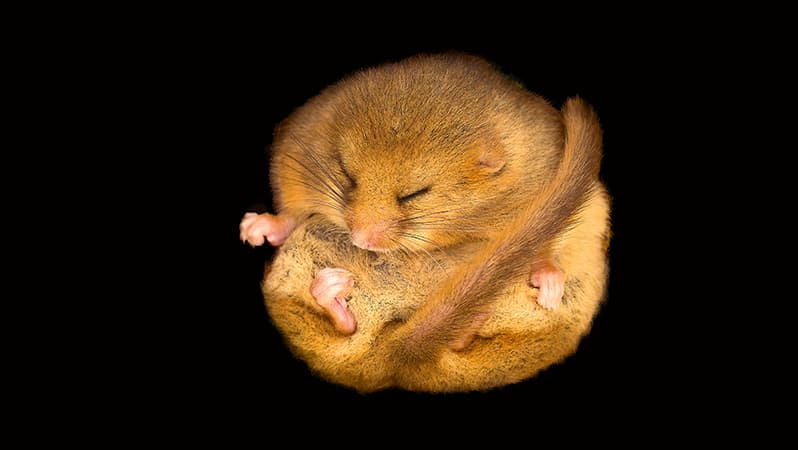
Hazel Dormouse. Green/Nature Picture Library/Getty Images.
DIMINISHING HOURS OF DAYLIGHT, decreasing temperatures and a reduction in available food all can trigger hibernation, which on a fundamental level alters the expression of numerous genes, including those that protect cells from damage and others that assist in breaking down body fat for fuel. Hibernation brings metabolism almost to a standstill. Body temperature, pulse, respiration and blood flow all fall during this shutdown.
In this state, the mammals must rewarm themselves every few days, drawing energy from stored fat in significant quantities. Scientists still don’t know exactly why these cycles of awakening happen, although they speculate that warming allows the animal to make needed cellular repairs that are impossible while sleeping. As temperatures rise and days lengthen, the animals wake up for good, skinny and starving, ready to find food and a mate. Hibernation ends as quickly as it began, reversing the physiological changes that occurred when they started hibernating. “A squirrel can go from freezing to normal in about two hours,” Martin says.
This predictable and orderly process stands in stark contrast to the biological chaos that ensues when a person suffers an ischemic stroke. When human brain tissues are suddenly starved of blood and the oxygen it carries, neurons respond by releasing large quantities of neurotransmitters. These send rapid-fire signals through the brain’s circuitry, setting off a chain of toxic events that can kill neurons even far from the site of the initial injury. “A tsunami of activity surges across the brain, sucking up oxygen it doesn’t have and leaving a trail of dead cells in its wake,” says Lee Schwamm, a neurologist and director of the Comprehensive Stroke Center at Massachusetts General Hospital. “The real problem is that there is insufficient blood supply to meet the demand.”
To limit the impact of a stroke and its aftermath, time is of the essence—treatment can succeed only if symptoms are recognized quickly and a patient can be rushed to a hospital equipped to treat stroke patients rapidly and well. “Simply put, time is brain,” says Ralph Sacco, chairman of neurology at the University of Miami and former president of the American Heart Association. To stop this cascade of destruction, physicians have three options—administer a drug called tissue plasminogen activator to break up the clot, remove it with clot retriever devices, or use both of the above treatments. These approaches can restore blood flow and a supply of life-saving oxygen to the brain. But the more time that has passed, the worse the ischemic and reperfusion injuries are when the blood returns, as the immune system unleashes immune cells called microglia that hurt healthy parts of the brain in their efforts to repair damaged tissue.
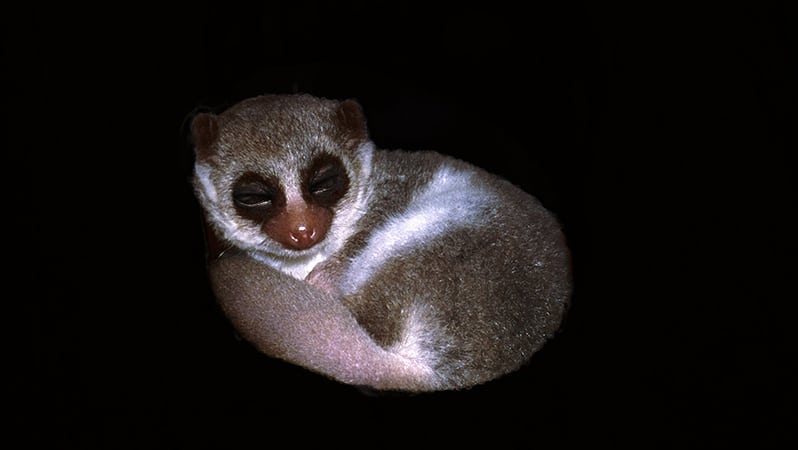
Madagascar’s Fat-tailed Dwarf Lemur. Werner Layer/Getty Images
COULD LESSONS FROM HIBERNATING animals help extend that golden hour? Perhaps, but only if researchers can pin down what exactly prevents those animals’ brains from starving for oxygen in the first place, or figure out what protects them from reperfusion injuries once the blood returns. In an effort to answer that second question, Drew’s team has spent nearly two decades hunting for protective mechanisms in a hibernator that underwent a stroke-like event.
She and her colleagues used a needle-thin probe to create tiny brain injuries—the closest simulation they could manage—both in Arctic ground squirrels that were hibernating and in those that were awake. Brain tissue in normal-temperature squirrels showed a cascade of damage and an inflammatory response in the tissue surrounding the probe, much like what happens to a person who has a stroke. But the intentional injury had significantly less impact in hibernating animals. Drew surmised that Arctic ground squirrels in this state are helped by some sort of neuroprotective compound.
Hjalmar Bouma, a pharmacologist and resident in acute internal medicine at the University of Groningen in the Netherlands, discovered what that compound might be—and is investigating it for clinical applications to protect against organ injury and conditions such as sepsis. As a medical student, Bouma had worked in the lab of Robert Henning, an anesthesiologist and pharmacologist at the university who was interested in hibernation as a way to protect organs during major surgery. For their experiments, Henning and Bouma used the Syrian hamster, a hibernating rodent native to the Middle East. The researchers compared hamster cells to kidney cells from rats as both sets of cells were chilled and rewarmed, simulating hypothermia and reperfusion.
The first clue about what set hibernating animals apart was olfactory. When Bouma and his colleagues opened the flask of the hamster’s cell cultures, “the liquid reeked of rotten eggs,” he says. Bouma guessed immediately that the smell meant the presence of hydrogen sulfide. All of those foul-smelling cells survived for 24 hours after being chilled and thawed, while only half of the rat kidney cells lived that long. When the researchers added hydrogen sulfide to the rat cells, the chemical blunted the harmful effects of reperfusion in those cells as well.
Bouma and others in Henning’s lab spent the next several years investigating how hydrogen sulfide was involved in hibernation. They knew from the work of other scientists that most mammals have only very small amounts of the compound, which acts as an antioxidant, counteracting the effects of “oxidative stress,” in which harmful chemicals wreak havoc on DNA, proteins and other important molecules. Not coincidentally in this context, oxidative stress also causes much of the damage during reperfusion after a stroke, adding to the effects of an overactive immune response. But Syrian hamsters and other hibernators, including 13-lined ground squirrels (a cousin of the Arctic ground squirrel), produce hydrogen sulfide in abundance, and Bouma hypothesized that its purpose could be to protect cells from oxidative stress.
Other studies added credence to that hypothesis. In her lab at the University of Wisconsin, Carey found evidence of oxidative stress in hibernating 13-lined ground squirrels. Her team also found that some cells in the hibernator’s body produce large numbers of protective proteins and reduce the synthesis of molecules that may increase stress to their cells—which may be part of the protection hibernators experience during the winter months.
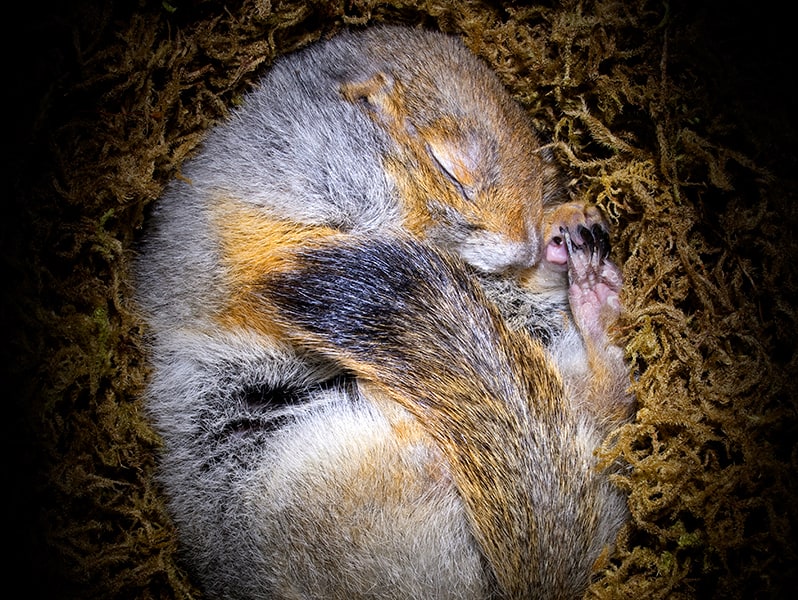
Arctic Ground Squirrel. Ingo Arndt/Minden Pictures/Getty Images.
CAN ANYTHING THAT HIBERNATING animals do be translated into strategies for preventing or treating stroke in people? Some of the first attempts took perhaps the most obvious approach—reducing a person’s body temperature to mimic the conditions under which hibernation occurs. But humans tend to shiver violently to maintain a normal body temperature, and shivering is a calorically expensive process that requires extra oxygen—just when, during a stroke, cells are oxygen-starved. Reducing body temperature thus puts an even greater strain on metabolism and could make stroke damage worse. Although hibernating animals do indeed experience hypothermia, that’s the effect of a slowed metabolism, not the cause.
A second possibility would be to imitate the immune system changes that occur during hibernation. Bouma, studying European ground squirrels, 13-lined ground squirrels and Syrian hamsters, found that levels of infection-fighting white blood cells dropped by more than 90% during hibernation because of retention in lymph nodes and adhesion to vessel walls. Moreover, the researchers also noted that these white blood cells have a reduced function, leading to a broader immunosuppression that affected all parts of the immune system and which may, in addition to the effect of hydrogen sulphide, serve to protect against organ injury.
Tamping down the immune system might also help protect human patients, suppressing the collateral damage caused by overenthusiastic microglia immune cells, which attempt to clean up a stroke injury and hurt healthy cells in the process, says Mihai Podgoreanu, an anesthesiologist at Duke University. “Preventing this secondary injury would be hugely helpful,” he says. But it could also leave patients at a higher risk of developing secondary infections.
Even if it were possible to isolate and reproduce some of the neuroprotective processes of hibernation—very large ifs at this point—Schwamm notes that “it is a leap of faith to think an injured human brain would have the same response as a 13-lined ground squirrel.” He points to the scientific literature on stroke, which is littered with failed clinical trials of drugs that appeared effective in animals but didn’t work in humans. Human brains are simply different, Schwamm says, and “the larger frontal lobes of people may simply not be amenable to hibernation.”
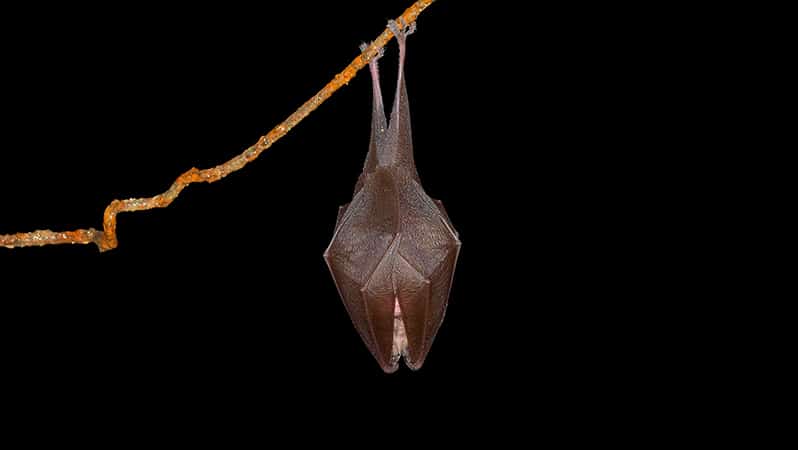
European Lesser Horseshoe Bat. Cucu Remus/Getty Images.
IN WHAT MAY BE the closest thing so far to a hibernation-inspired stroke therapy, John Hallenbeck, chief of the clinical investigations section of the National Institute of Neurological Disorders and Stroke, and Yang-ja Lee, his co-investigator, in November 2017 identified a potential drug that might help protect human brains.
Their discovery involves a cellular process called SUMOylation, which tags proteins with small molecules that, among other things, direct the proteins to their correct locations in a cell and alter how readily they can be recycled. SUMOylation modulates a variety of biochemical pathways, and this wide-ranging activity is part of what scientists think makes SUMOylation so advantageous. Indeed, previous studies showed that increasing SUMOylation in cells could protect them against damage from ischemia, a lack of blood flow and oxygen. Because they had observed that this beneficial process goes into overdrive in the brains of the hibernating squirrels, Hallenbeck and Lee wondered whether SUMOylation might be part of what keeps the animals from being harmed by the stroke-like conditions of hibernation.
Their next step was to look for a compound that could spur SUMOylation in human brains and perhaps limit the damage of a stroke. Hallenbeck and Lee used an automated process to comb through a library of more than 4,000 molecules. They found compounds that increased expression of the SUMOylating enzyme, and some that inhibited an enzyme removing such tags, including a selenium-containing molecule called ebselen that boosted SUMOylation in cultured rat neurons. When the researchers injected ebselen into the brains of healthy mice, the molecule enhanced SUMOylation and appeared to reduce the number of neurons that died from ischemia.
“For stroke treatment, it seems that studying hibernation was a good strategy,” says Lee. The researchers now hope to replicate these results in human neurons.
A follow-up study published in January 2018 by Lee, Hallenbeck and colleagues revealed a potential mechanism for extreme ischemia tolerance in ground squirrels in which their brain cells cycled between two different biochemical states, giving them an extreme tolerance to stress.
Meanwhile, Bouma’s and Henning’s group in the Netherlands is beginning preliminary trials using treatments based on the effects of hydrogen sulfide to prevent acute kidney injury—during critical illness and major cardiac surgery. And Drew’s team at the University of Alaska is starting its own trials. After several decades of exploring the processes of hibernation, Drew has homed in on the A1 adenosine receptor, a protein that is present throughout the bodies of ground squirrels, humans and other animals. Drew’s previous work had flagged the receptor as a potential hub of hibernation, and when she activated it in Arctic ground squirrels, the rodents’ body temperatures plunged far below normal. That makes the A1 adenosine receptor possibly useful as part of a stroke treatment, but activating it can also trigger dangerous heart arrhythmias. Now Drew is searching for molecules that can stimulate the A1 adenosine receptor in humans without creating cardiac problems.
Even as scientists begin to experiment with ways to use the processes of hibernation to treat strokes in people, most mysteries about how it works in animals remain unsolved. Still, those who are involved in this research have high hopes that greater understanding will come, and with it the ability to harness hibernation’s power to protect human brains from the ravages of ischemia. Says Duke anesthesiologist Podgoreanu, “Maybe it’s time we let Mother Nature inform us how things can be done.”
Dossier
“Induction of Torpor: Mimicking Natural Metabolic Suppression for Biomedical Applications,” by Hjalmar R. Bouma et al., Journal of Cellular Physiology, May 26, 2011. This paper provides an overview of how scientists are planning to create a hibernation-like state in individuals with ischemia and other types of injuries.
“Neuroprotection: Lessons from Hibernators,” by Kunjan R. Dave et al., Comparative Biochemistry and Physiology Part B: Biochemistry and Molecular Biology, February 2012. In this review, scientists discuss adaptations in mammals that may suggest therapeutic targets and strategies to protect the human brain.
Winter World: The Ingenuity of Animal Survival, by Bernd Heinrich, HarperCollins Publishers, 2003. This book examines the clever strategies animals use to survive frigid temperatures, including hibernation
Stay on the frontiers of medicine
Related Stories
- A Shake-Up in Stroke Treatment
A new approach for restoring blood flow to the brain is having unprecedented success.
- Why Strokes Still Kill
Terrible things happen fast in a victim’s brain. Now new drugs and smart systems can extend the treatment deadline.