Published On March 28, 2022
The precise origin of COVID-19 is still uncertain. But whether it came from a bat or not, the virus now passes freely between humans and animals—pigs, rabbits, tigers, bats, white-tailed deer, mink, house cats, bank voles, ferrets and gorillas, to name a few. The pandemic crosses borders not only between nations, but barriers between species, so efforts to contain its spread will have to account not only for human politics but also managing ecosystems and populations of wild animals—where the disease can reside, evolve and emerge again as a lethal threat.
In that sense, COVID-19 joins the majority of known infectious diseases, about 60% of which can spread to and from nonhuman animals. These so-called zoonotic diseases, a long-standing burden, are a problem that keeps growing. “Over the past 50 or 60 years, newly recorded zoonotic viruses have been spilling over to humans at a pretty constant rate of about two per year,” says Andy Dobson, a professor of ecology and evolutionary biology at Princeton University who studies wildlife diseases. “But the proportion that create epidemics and pandemics is increasing.” About three out of four new infectious diseases now come from animals.
“Emerging zoonotic infectious diseases are here to stay,” says Casey Barton Behravesh, director of the One Health Office at the Centers for Disease Control and Prevention. One Health began more than a decade ago as an international effort to look at a wide range of health-related interactions between humans, animals and environments. “Preventing future outbreaks requires an approach that includes collaboration across the human health, animal health and environmental spectrum,” Behravesh says.
This idea of stewardship of global ecology stands in stark contrast to the current pandemic response, which has focused on what to do after a virus spreads widely. Dobson and other experts argue that rather than spend vast sums trying to contain a global contagion, the nations of the world should make investments in “safeguarding nature” to prevent zoonotic pandemics from occurring in the first place. In a February 2022 article in Science Advances, Dobson and other members of a global task force argued that targeting some causes of disease spread, such as deforestation and the wildlife trade, could cost as little as $22 billion a year—or about 2% of the economic and mortality costs of the COVID-19 pandemic, which economists predict could reach $10 trillion to $20 trillion.
But it’s not only new diseases that pass to people from the animal kingdom. Researchers also continue to be concerned about endemic vector-borne diseases such as malaria and dengue, which refuse to be eradicated. Climate change and other factors, such as busy global travel routes, have enlarged the natural ranges of those diseases’ host species and made them more of a threat than ever. Dengue cases alone have increased more than eightfold over the past two decades, says Dobson, and “more kids still die every day from malaria than people who were killed in 9/11.”
International consensus moves slowly, however, and expensive global initiatives are likely to be a hard sell. A more feasible approach may be to target the natural world tactically. Such interventions must avoid the tragic mistakes of DDT, which destroyed entire ecosystems in the effort to kill the mosquitoes that carried malaria. Today, researchers are pursuing gentler approaches to block diseases in the animals before they can transmit them to humans.
“It’s going to take different forms of control,” says Dobson, “because the things we currently have to kill with—insecticides and poisons—aren’t specific enough. We need more creative ways of working with nature, more creative solutions and less of a despotic, ‘kill everything’ mindset.” The past few years have delivered several landmark advances that may fit that bill. Taken together, innovations in vector management could slow the advance of zoonotic disease, a desperately needed step.
The Aedes aegypti mosquito is the primary vector for Zika, chikungunya and dengue, a flavivirus that causes flu-like symptoms in about 96 million people a year. Because Ae. aegypti mosquitoes live in urban and semi-urban environments throughout the tropics and subtropics, they’ve been especially hard to control with insecticides. Those chemicals can affect other species too, including humans, and when they do reach their targets, mosquitoes are often quick to build up immunity. “Driving up and down the street with trucks of fogging insecticides is largely a waste of time,” says Scott Ritchie, a medical entomologist and principal investigator in the Bill & Melinda Gates Foundation–funded Eliminate Dengue program.
Bats help in human ecosystems, so efforts should target the diseases, not the animals.
Ritchie and his colleagues at the nonprofit World Mosquito Program, led by medical entomologist Scott O'Neill, have experimented with an alternative approach. They found that a promising bacterium of the Wolbachia genus can be introduced into mosquito colonies as its own epidemic. Infection with this pathogen blocks the insects’ ability to transmit dengue. In one of the first large-scale field tests of the technology, Ritchie and other researchers introduced a total of 4 million Wolbachia-infected Ae. aegypti mosquitoes to insect populations in and around Cairns and Townsville, Australia. Soon, almost all of the local target mosquitoes had the bacteria, and there has been almost no local transmission of dengue since the program’s launch in 2013. The approach was then tried in Yogyakarta, Indonesia, which saw a 77% drop in reported cases.
Ritchie and his team infect mosquitoes with a strain of Wolbachia called wMel. Although it doesn’t naturally infect Ae. aegypti, the wMel strain is able to take up residence in those mosquitoes in an arrangement called endosymbiosis. Inside the insects’ cells, wMel competes for nutrients that the dengue virus needs for replication. “They still get infected and hold virus in their gut, but the amount in their salivary glands is significantly reduced, so that can't transmit it,” says Ritchie.
A much bigger prize would be malaria, which is transmitted to humans through the bite of female Anopheles mosquitoes and kills more than 600,000 people annually, according to the World Health Organization. So far, however, progress has been limited. In 2013, researchers at Michigan State University led by Zhiyong Xi established the first stable line of Wolbachia-infected Anopheles stephensi—a common malaria vector in urban India. Although those infected mosquitoes were too weak to spread effectively through wild populations, Xi and other teams have since managed to introduce the bacterium into two additional Anopheles species that cause malaria in Africa.
A controversial innovation called a gene drive might eventually help turn malaria’s tide. Instead of introducing an infection, as in the Wolbachia approach, the gene drive uses CRISPR gene-editing technology to inhibit a mosquito’s ability to reproduce or to carry the parasite that causes malaria. Programmable guide RNA is inserted into the genome of a mosquito, and as the gene-drive mosquitoes breed with others, that genetic variant spreads through the population by capitalizing on the “selfish” quality of some genes.
A BETTER NEIGHBOR TO NATURE
The One Health initiative advocates a comprehensive global approach.
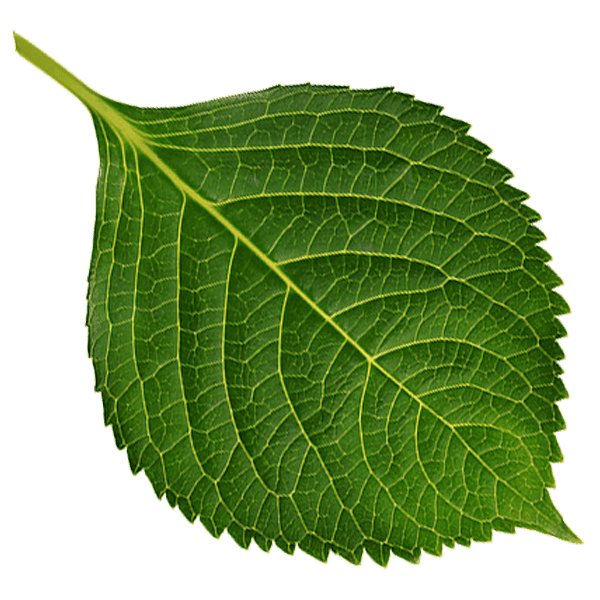
Respecting Habitats
Encroaching on wild spaces makes outbreaks more likely.
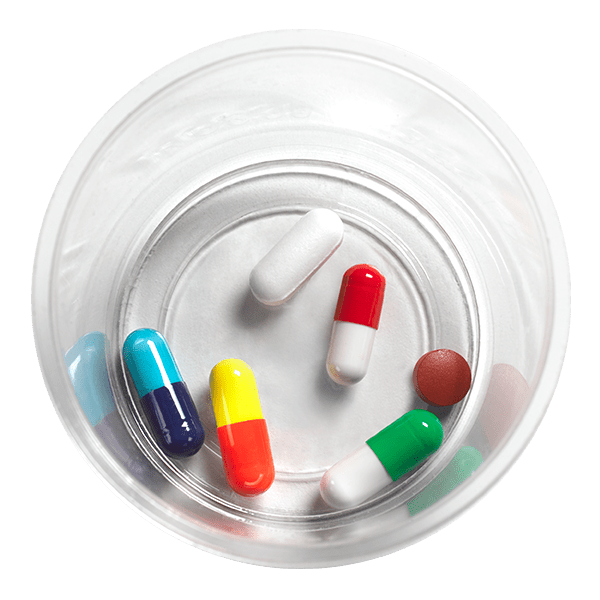
Fewer Antibiotics
Dialing back the use of these in livestock leads to fewer drug-resistant bacteria in humans.
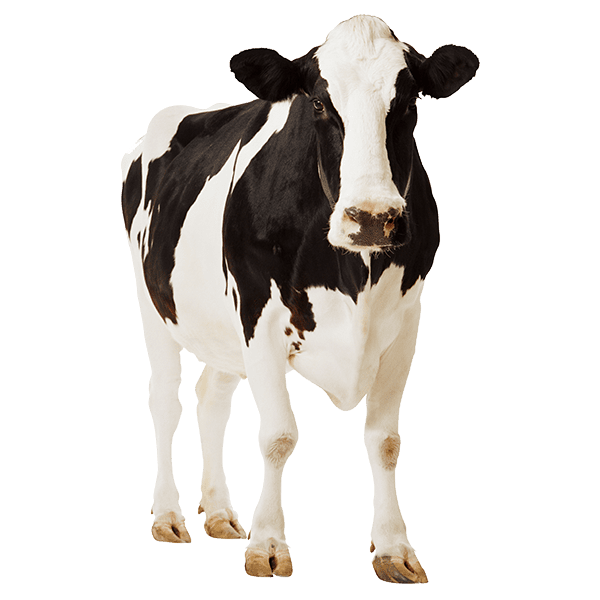
Tighter Surveillance
Monitoring livestock and wildlife can spot pathogens earlier.
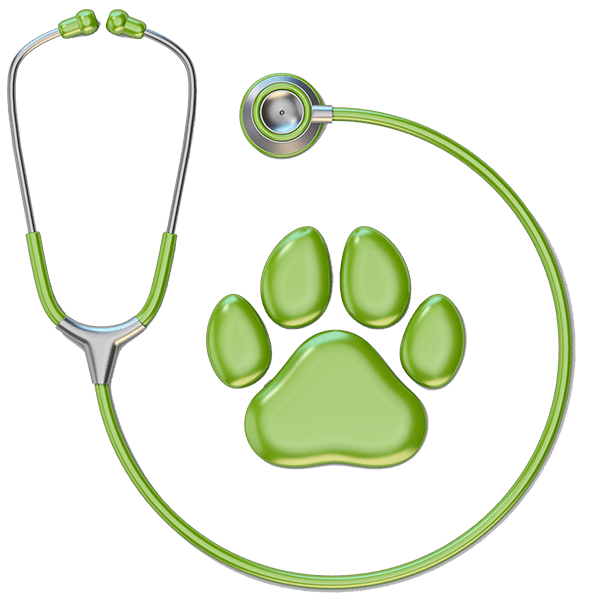
More
Vets
Animal health specialists are especially critical in Africa, but their numbers are currently low.
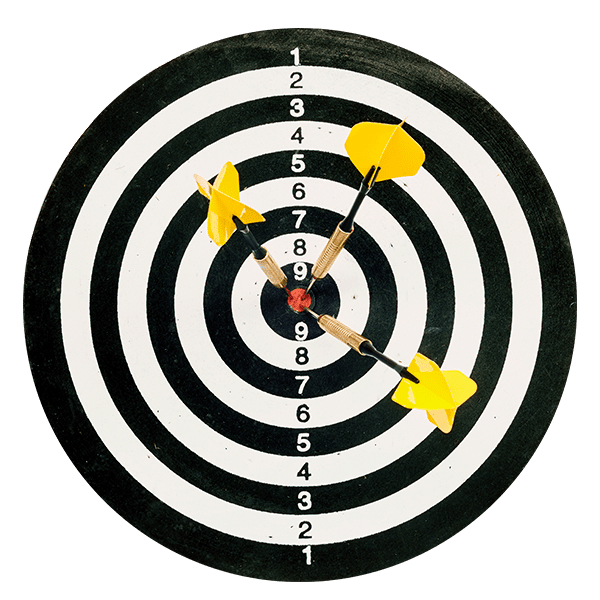
Disease Management
New tools that target disease, not carriers, are the goal.
In nature, some genes improve on the 50-50 odds that they’ll be inherited by offspring. The CRISPR edit mimics this process using a construct on one chromosome. During an early stage of development, the CRISPR part of the gene drive finds and cuts out the normal gene on the opposite chromosome. The gene drive then serves as a template when that cut is repaired, resulting in a mosquito that now carries two copies of the modified gene, bringing the inheritance rate to nearly 100%.
While gene drives might be used to reduce or wipe out wild populations of mosquitoes, in some cases that might simply clear out an ecological niche for a worse vector. Instead, scientists from the University of California, Irvine, UC Davis and Federal University Oye-Ekiti in Nigeria recently tested a gene drive that blocked transmission of the malaria parasite in Anopheles gambiae. Within six generations, or about six months, every mosquito had at least one copy of the gene-drive construct. That’s well within one annual malaria transmission cycle and suggests that gene drives might ultimately be part of an effective control strategy.
Long before bats were investigated as the first vector for COVID-19, they were known for transmitting other diseases, including rabies. Yet bats also do good work—for example, by devouring insects at a rate that would otherwise require at least $3.7 billion in annual pest control costs in the United States, according to the National Park Service. Bats are also important as pollinators and seed spreaders, which means that targeting disease in bats should do its utmost to protect the bats themselves.
In Mexico and Latin America, vampire bats (Desmodus rotundus) are the principal spreaders of rabies, and topical anticoagulant poisons, which spread from bat to bat, are frequently used. But these poisons might affect the wider environment, and mathematical modeling suggests that it would require intensive, coordinated culls across impractically large areas to reduce rabies rates.
Since the early 2000s, researchers have studied whether it was possible to vaccinate the animals instead. Topical oral vaccines could spread to members of a colony in much the way that poisons are currently transmitted. “Vampire bats are highly social and they do a lot of grooming of each other,” says Daniel Streicker, a Wellcome Trust senior research fellow in the Institute of Biodiversity, Animal Health and Comparative Medicine at the University of Glasgow in Scotland. His lab is currently exploring two types of “self-spreading” vaccines for inoculating bats: transferable vaccines, which spread only from treated bats; and transmissible vaccines, which can spread infectiously through multiple generations.
This vaccine uniquely targets the carrier, not the disease.
In a 2019 paper in Nature Ecology & Evolution, Streicker and his fellow researchers from the University of Michigan used field experiments and mathematical modeling to show that a transferrable topical vaccine could spread at high enough rates to inoculate a large fraction of a bat colony. At locations in Lima, Peru, they captured roughly a quarter to a third of bats in colonies of 200 to 250 animals. They applied a gel containing a biomarker that shows up as bright-orange fluorescence in the animal’s fur once it’s ingested, and then released the affected bats. At the end of four weeks, an estimated 84% of bats in one colony showed the fluorescent marker, and 92% of bats in another had it.
Streicker believes his group’s work on transmissible rabies vaccines, still at an earlier stage than the development of transferable vaccines for bats, could ultimately help limit the spread of other diseases such as Nipah virus and Hendra virus that infect humans on a periodic, recurring basis. Such a vaccine could provide lasting immunity for bat populations, and new vaccines could be developed as potentially dangerous diseases appear.
Deer ticks are the agents through which the bacterium Borrelia burgdorferi infects humans with Lyme disease, a potentially debilitating illness that is still incompletely understood. In the United States, Lyme disease is the most common infection transmitted from animals to humans, and reported cases have nearly doubled since 2004.
Erol Fikrig’s laboratory at Yale University School of Medicine wants to address the problem by implementing a new kind of vaccine. Vaccines generally work by priming a body to repel the virus or bacteria that invades the body and causes harm. Fikrig’s project targets the vector—the tick itself—rather than the pathogen it carries, an approach that could be game-changing for zoonotic disease.
When a vector animal bites someone, it can sometimes add a cocktail of salivary compounds—anticoagulants or anti-inflammatory agents—that bypass the skin and a local immune response. In deer tick saliva, for instance, Fikrig’s group identified 19 key proteins. His mRNA vaccine—the same kind of vaccine that has proved so successful in protecting against COVID-19—trains the body to fight those proteins that help the B. burgdorferi bacterium take hold. (B. burgdorferi itself has proven resistant to vaccine development.)
The result is that the body reacts more vigorously to the tick’s bite, making it harder for infection to occur. In a guinea pig study published in Science Translational Medicine in November 2021, bites of vaccinated animals became red and inflamed within 18 hours, alerting them to the tick. Because Lyme bacteria can take a day or two to be transmitted, this early warning could be a game changer in humans by alerting them to a tick bite that might normally go undetected.
The study also found that ticks didn’t hang on to vaccinated guinea pigs for as long. “Normally, ticks feed for four to five days on a guinea pig,” says Fikrig. “These ticks seem to come off at about two days, and their weights were lower. It’s like they took a little meal and maybe were nauseous, and just gave up.It’s a slow-transmitting pathogen, so if we can really change the feeding behavior, we have the capacity to prevent transmission,” Fikrig says.
Fikrig says the same kind of vaccine could also help with other slow-transmitting tick-borne diseases, including babesiosis, a rare but occasionally life-threatening disease found in the United States. The current vaccine would be unlikely to work against others, such as the emerging Powassan virus, which is transmitted from tick to host in about 15 minutes. There is also more basic science to do, says Fikrig, who notes that the current vaccine worked in guinea pigs but not in mice, and thus might not yet be effective in humans.
Indeed, while new barriers against animal-borne disease are sorely needed, caution has been the watchword, especially when efforts have ventured into new territory. Forests are not hospitals, and researchers are keen to avoid unintended consequences that they cannot be on hand to oversee. Gene drives in particular, because they send a cascading genetic change through wild populations, have raised biosafety concerns, and environmental groups and governments have issued strong warnings about their use. Proponents of the technology now are seeking to develop new strategies and safeguards.
Some vaccines for bats have been tested in captive environments, and researchers say they might be ready for use in the wild in another five years or so. Other bat vaccines that spread infectiously between populations could be 10 years away. Nonetheless, the University of Glasgow’s Streicker sees his lab’s work as part of a fundamental shift in approach—away from trying to manage emerging diseases only after they’ve begun to infect livestock or humans. “Now we think we can intervene earlier in that process,” Streicker says, “and instead of just killing these animals, we may be able to make them less effective transmitters of diseases that we don’t want to have jumping into people.”
Dossier
CDC.gov/onehealth/zohu, Zoonoses and One Health Updates. One-hour monthly webinars from the Centers for Disease Control and Prevention cover emerging health threats at “the animal-human-environment interface.”
“Epidemiology and biology of a herpesvirus in rabies endemic vampire bat populations,” by Megan E. Griffiths et al., Nature Communications, November 2020. The researchers look at a transmissible rabies vaccine for vampire bats, using a betaherpesvirus as a vaccine vector.
“Gene Drives Gaining Speed,” by Ethan Bier, Nature Reviews Genetics, August 2021. This review paper describes how gene-drive systems work, different types of gene drives and potential strategies for their deployment.
Stay on the frontiers of medicine